Filtration of infectious aerosols
The airborne transmission of respiratory pathogens in indoor environments and associated respiratory illnesses lead to large excesses in expenses associated with health care, absence from work, and lost worker productivity. However, the transport and control of airborne infectious diseases in indoor environments remains poorly understood. Several studies have shown that building-related factors such as increased outdoor air ventilation rates, lower occupant density, and use of UV germicidal irradiation can reduce the risk of infectious disease transmission inside buildings, but because each of these mechanisms works to remove or inactivate airborne infectious particle concentrations indoors, might commonly available particle filters in recirculating heating, ventilating, and air-conditioning (HVAC) systems also reduce the risk of infectious disease while potentially requiring less energy to do so?
To help address this question, this work (1) reviewed existing literature on the physical, biological, and epidemiological factors that influence the transmission of airborne infectious diseases; (2) connected relevant findings from the review and a standard Wells-Riley risk model to existing knowledge of HVAC particle filtration standards (i.e., MERV from ASHRAE Standard 52.2); and (3) described and applied a modified Wells-Riley methodology for estimating the impact of commonly available HVAC filtration on the spread of infectious disease in buildings using individual case studies of airborne influenza, rhinovirus, and tuberculosis transmission in hypothetical office, school classroom, and hospital waiting room environments designed to meet ASHRAE Standard 62.1 as a baseline.
On average across the 9 case studies modeled herein, MERV 4, 7, and 11 filters are expected to provide reductions in the risk of infection from one infected individual of approximately 20%, 50%, and 60%, respectively. MERV 13-16 and HEPA filters are all predicted to provide risk reductions of approximately 64-67%. Using a range of assumptions for HVAC system operation, building occupancy, filter costs and replacement schedules, and energy costs in four U.S. climates (including Chicago, IL, Charlotte, NC, Houston, TX, and Phoenix, AZ), all HVAC filtration products with the exception of MERV 4 were estimated to provide these risk reductions at a lower cost than an equivalent amount of outdoor ventilation in each hypothetical environment. An example cost-risk comparison for the office environment is shown to the right. Overall, HVAC filtration products are predicted to achieve the greatest risk reductions at lower costs of operation relative to providing an equivalent amount of outdoor air ventilation.
Read the full report for the NAFA Foundation.
After completion of the NAFA Foundation report, we continued to revise our model and narrowed our focus to influenza transmission alone.
Detailed methods for influenza modeling
When an individual coughs, sneezes, speaks, or breathes, droplets consisting of water, proteins, salts, and various organic and inorganic matter are expelled into the air. If the emitter has a respiratory infection, those emitted droplets can also contain smaller infectious bacteria or virus particles themselves, depending on the type of infection. Once expelled from the high relative humidity environment of the human body to a relatively less humid indoor environment, droplets rapidly decrease in size as the surrounding liquid evaporates. The largest of the emitted particles (called “droplets”, or particles greater than 5 or 10 µm) remain suspended only for a short period of time in indoor environments. Large droplets can lead to very short-range transmission by inhalation or, alternatively, can deposit onto hands or inanimate objects, which can then lead to “fomite” transmission whereby deposited bacteria or viruses particles are transferred directly to mucous membranes of the eyes or nose by skin contact. The smallest of the emitted particles (called “droplet nuclei”, or particles smaller than about 5 µm) can travel farther from the source and can ultimately be inhaled into a susceptible person’s lower respiratory tract. Although the relative contribution of fomite, respiratory droplet, or respiratory droplet nuclei transmission for many infectious diseases is poorly understood, we do know that airborne transmission has been shown to be a predominant route of transmission for a number of communicable diseases, including both rhinovirus and influenza. Importantly, as the ASHRAE Position Document on Airborne Infectious Diseases discusses, if an infectious disease is transmitted via the airborne route, HVAC engineers can likely play a role in designing control systems to reduce concentrations of droplet nuclei and lower the risk of disease transmission. And critical to specifying many control strategies, including HVAC particle filtration, is accurate knowledge of the particle sizes of infectious aerosols that exist in indoor environments (see figure below).
It is commonly believed that droplet nuclei particles average 1 to 3 µm in diameter, although some studies have shown considerable variation in the size distribution of expelled droplets and droplet nuclei particles. Several recent studies utilizing more advanced measurement techniques than earlier investigations have revealed a general consensus that the majority of particles expelled during various human airway activities (often 80-90%) are actually smaller than 1-2 µm in diameter. However, it may also be more appropriate to consider in what particle size fractions viruses and bacteria are actually contained.
Several more recent studies have utilized more sophisticated techniques (e.g., quantitative polymerase chain reaction, or q-PCR) to identify the presence of viruses or bacteria in expelled droplets and droplet nuclei collected on multi-stage bioaerosol samplers. Most of these studies have focused on the particle size distributions of influenza in stationary and personal airborne particulate matter samplers deployed in indoor environments, including healthcare centers, daycare centers, hospital emergency rooms, simulated patient rooms, as well as in the coughing/breathing zone of both people and manikins. These studies offer insight not only into what size aerosols exist after expulsion from the human body, but in what particle size fractions are viruses or bacteria actually present and are thus likely of most concern for infectious disease transmission.
For the purposes of informing HVAC engineers in designing control strategies for infectious disease transmission, it is important to note that the particle size bins from recent studies align relatively closely to the three size bins outlined in the primary metric of the most commonly used HVAC filtration standard in the U.S: the Minimum Efficiency Reporting Value (MERV) as described in ASHRAE Standard 52.2. The MERV classification system categorizes filters and other air-cleaning devices by their particle removal efficiency of 0.3-1 µm, 1-3 µm, and 3-10 µm particles. Therefore, in an attempt to reconcile the reported particle size distributions of influenza virus in airborne particulate matter in the aforementioned studies with MERV, the fractional distributions of influenza from our literature review were adjusted to fit within the three particle size ranges in Standard 52.2 by simply assuming that the virus content has a uniform distribution in each particle size bin. These assumed distributions are shown in the figure below for each reported study, as well as the average across all studies and sampling locations. Although this assumption may suffer from large uncertainty, the magnitude of error is unknown at this time.
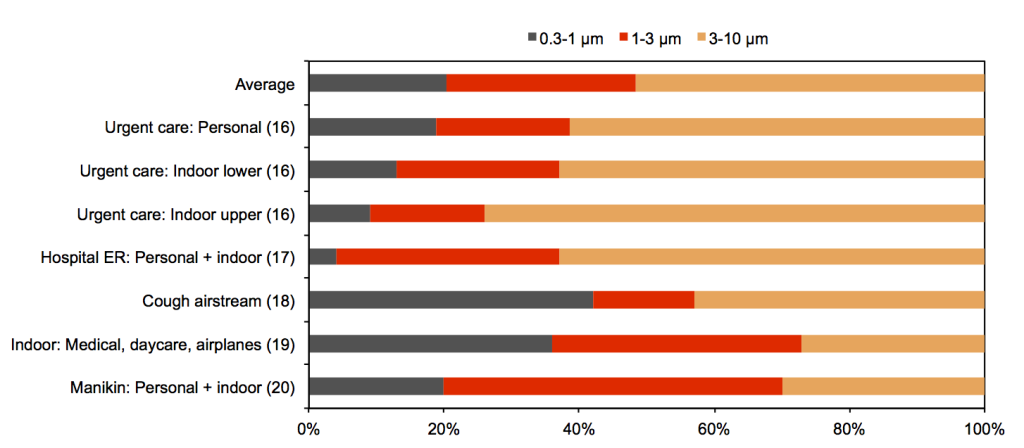
Estimated fractions of influenza virus found in 0.3-1 µm, 1-3 µm, and 3-10 µm particles in five recent studies of indoor environments
On average across all of these studies, approximately 20% of influenza virus particles are estimated to be associated with particles in the 0.3-1 µm size range; 29% are estimated to be found in the 1-3 µm size range; and 51% are estimated to be associated with the 3-10 µm size range. The smallest size range has the highest deviation from the mean, followed by the middle size range, and then the largest size range.
We then connected these assumptions for particle size distributions to MERV to estimate filtration efficiency for influenza aerosols:
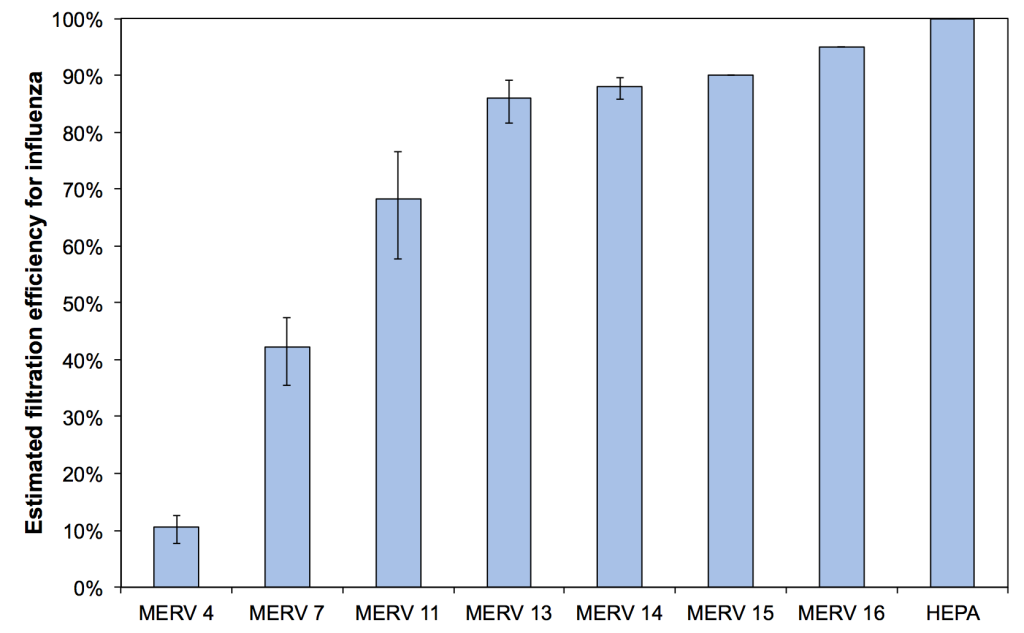
Estimated mean, minimum, and maximum filtration efficiencies for airborne influenza in indoor environments
Once filtration efficiency values for influenza were estimated, we modeled the impact that HVAC filtration may have on risks to individuals in an indoor environment. We used a modified version of the well-known Wells-Riley equation to account for particle size effects and deposition and filtration. We used this model to predict the impact that filtration could have on risks of influenza transmission in a hypothetical office building. We also compared estimates of the cost of filtration versus the costs of an equivalent amount of outdoor air ventilation in a range of U.S. climates. The figure below shows the predicted risk of infection by the influenza virus during an 8-hour workday in the hypothetical office building using a range of HVAC filters installed in the constant operation HVAC system, from no filter to HEPA.
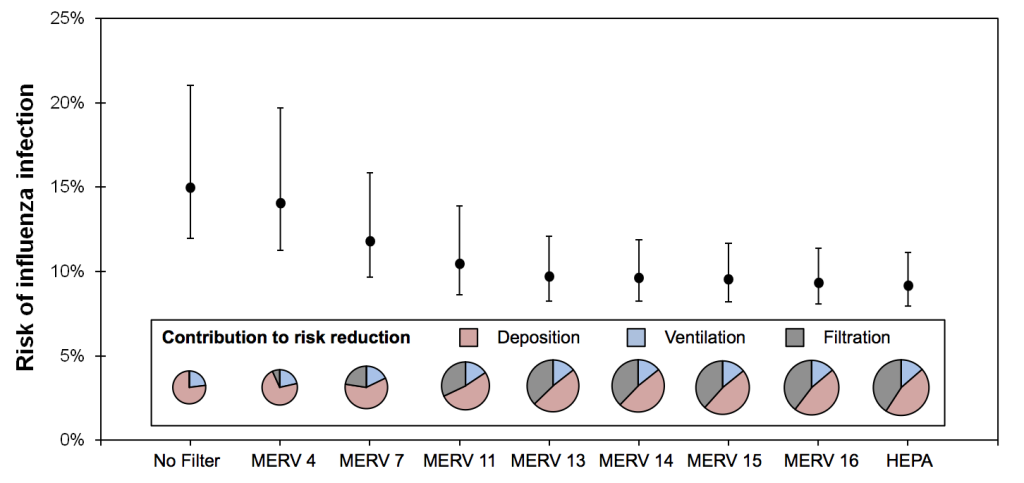
Predicted risk of infection by influenza virus in the hypothetical office environment with various levels of HVAC filtration installed, along with contributions of deposition, ventilation, and filtration to risk reductions (note that the diameter of the pie charts scale with total virus loss rate)
Finally, the figure below plots the mean predicted relative risk (RR) values from the Wells-Riley modeling (based on estimates from the figure above, assuming no filtration as the baseline condition) versus estimates of the total cost of providing that same risk reduction with both outdoor air ventilation (using mean values across the four cities) and with each level of HVAC filtration. To compare OA ventilation in each location directly to HVAC filtration, OA ventilation rates were adjusted to achieve the same removal rate as that of each level of HVAC filtration. For example, a MERV 13 filter is expected to achieve 1.31 per hour of influenza aerosol removal in the office environment with the mean assumption for infectious particle size distributions; for outdoor air ventilation to achieve the same risk reduction as filtration, the office HVAC system would need to provide an additional 1.31 air changes per hour of outdoor air ventilation.
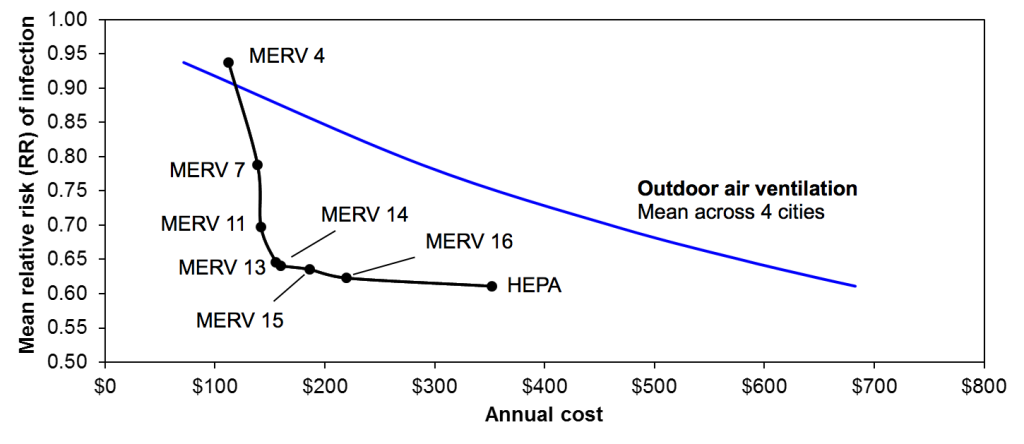
Relative risk (RR) of influenza transmission in the hypothetical office environment with both HVAC filtration and equivalent outdoor air ventilation rates
Besides MERV 4, which has a very low infectious particle removal efficiency, HVAC filtration is predicted to be less expensive on annual basis in comparison to outdoor air ventilation in each climate for each level of risk reduction. MERV 13 and MERV 14 filters appear to achieve an optimal combination of lowest risk reductions at least costs. MERV 4 is actually more expensive to operate than providing equivalent outdoor air ventilation in each climate, largely because of the very low effectiveness in controlling infectious aerosols. HEPA filtration appears to offer only a small incremental advantage over MERV 13-16 filters (only 1-4% lower RR) for approximately 1.5-2 times the cost of operation, which suggests that MERV 13-16 filters may be most appropriate for cost-effectively reducing risks of influenza transmission in this case study environment. In fact, switching from MERV 7 to MERV 13 is predicted reduce the number of infected individuals in this office environment by one person at an additional annual cost of only $17. Compared to the estimated economic gains of preventing a single influenza case of approximately $375, MERV 13 filtration could provide a benefit-to-cost ratio of 20 or more. These basic relationships were not sensitive to the various assumptions for infectious particle size distributions.
Read the article published on this work, accepted to Building and Environment in August 2013.
Acknowledgments
We are grateful for the National Air Filtration Association (NAFA) Foundation for sponsoring this project. Parham Azimi was the lead graduate student on this work.